By the Domainex Synthesis Group (Hugh Tawell, Andrew Jones, Andrea Bombana, Venu Komanduri, James Harnedy, Brahmam Medapi, Robyn Presland, and Vinny Duong)
In this edition of our blog series, we have focused on the following two recent publications, which describe interesting and useful synthetic transformations:
- An Air-Stable, Single-Component Iridium Precatalyst for the Borylation of C–H Bonds on Large to Miniaturized Scales. Kyan A. D’Angelo, Chris La, Brian Kotecki, Jake W. Wilson, Caleb Karmel, Rafal Swiatowiec, Noah P. Tu, Shashank Shekhar, John F. Hartwig, J. Am. Chem. Soc., 2024.
- Photochemical Permutation of Thiazoles, Isothiazoles and other Azoles. Baptiste Roure, Maialen Alonso, Giovanni Lonardi, Dilara Berna Yildiz, Cornelia S. Buettner, Thiago dos Santos, Yan Xu, Martin Bossart, Volker Derdau, María Méndez, Josep Llaveria, Alessandro Ruffoni, Daniele Leonori. Nature, 2024.
An Air-Stable, Single-Component Iridium Precatalyst for the Borylation of C−H Bonds
Organoboron compounds are highly valuable in organic chemistry due to their ability to participate in cross-coupling reactions and their synthetic versatility. Developing effective methods for synthesising borylated compounds is thus essential. Among these, the borylation of aryl C–H bonds is a widely adopted strategy, converting C–H bonds into C–B bonds using an iridium catalyst, an N,N-ligand, and bis(pinacolato)diboron (B2pin2) (Scheme 1). However, this methodology has notable drawbacks, primarily the instability of the widely used iridium precursor [Ir(cod)OMe]2. This instability can lead to irreproducible results, especially at large industrial or small experimental scales.
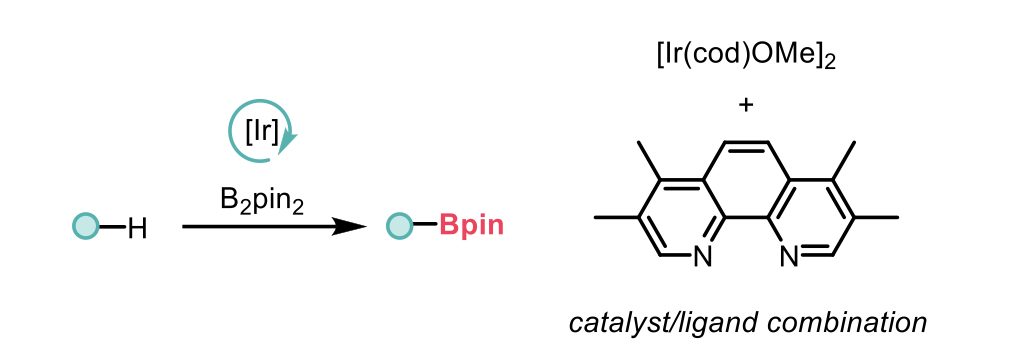
Scheme 1: Traditional C-H borylation schematic
To address these limitations, the authors of this study designed and synthesised [(tmphen)Ir(coe)2Cl], a single-component, air-stable iridium catalyst (Figure 1). This system activates upon the simple addition of arene, B2pin2, and solvent, requiring no additional additives. It offers activity and selectivity comparable to traditional two-component systems while functioning across a wide range of scales, from decagrams to micromoles or nanomoles in high-throughput experimental setups.
[(tmphen)Ir(coe)2Cl] functions as an effective single-component precatalyst that generates the active iridium(III) tris(boryl) complex under mild conditions. This new catalyst eliminates the need for air-sensitive additives or complex preparation protocols. Structural studies revealed that its design—pairing a weakly electron-donating chloride ligand with tmphen (3,4,7,8-tetramethylphenanthroline)—confers both air stability and high catalytic activity. The catalyst proved robust, retaining full activity even after a year of air exposure.
![Newly developed precatalyst system [(tmphen)Ir(coe)2Cl]](/sites/default/files/inline-images/Figure%201%20%5B%28tmphen%29Ir%28coe%292Cl%5D.png)
Figure 1: Newly developed precatalyst system [(tmphen)Ir(coe)2Cl]
The precatalyst successfully borylated a diverse range of arenes and heteroarenes, including challenging substrates like indoles, pyridines, thiophenes, and pyrroles. The methodology extended to unreported bioactive compounds such as ticlopidine, achieving good selectivity. These reactions were performed in both glovebox and ambient air conditions, highlighting the system’s reliability and robustness.
Crucially, the precatalyst demonstrated consistent results at both 10 mmol and 10 μmol scales, confirming its versatility as a scalable, drop-in replacement for the inconsistent traditional iridium catalyst systems.
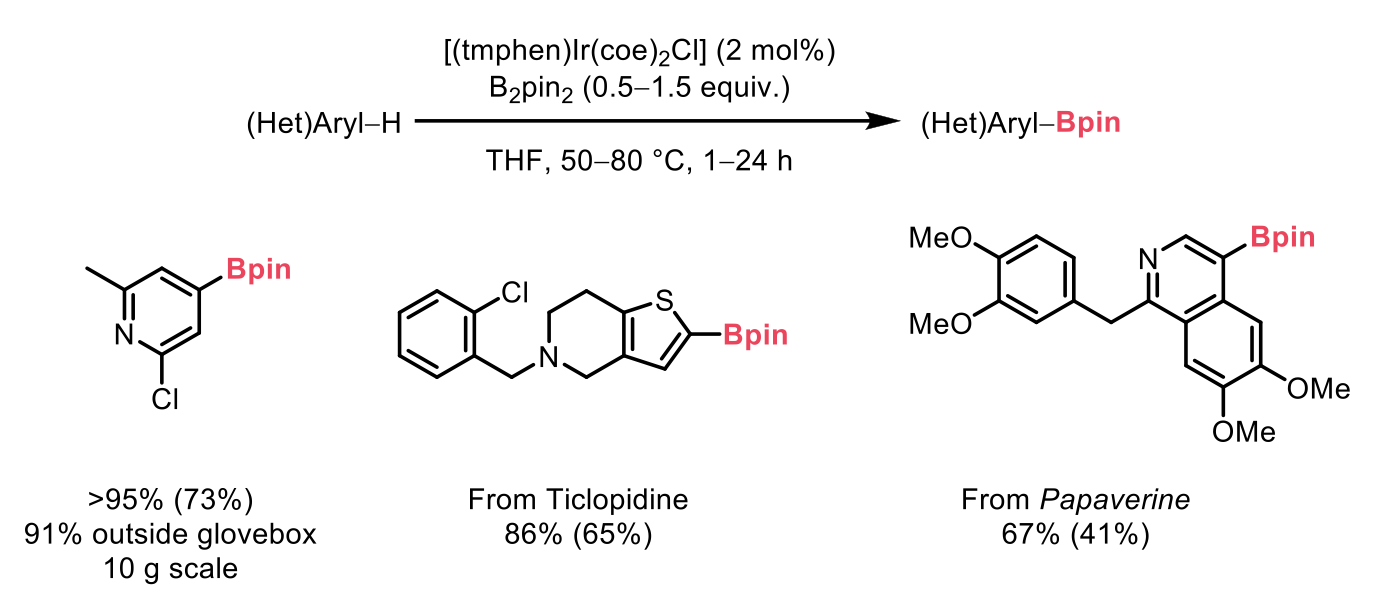
Scheme 2: Examples of C-H borylation substrate scope. 1H NMR yields are reported first and isolated yields in parentheses for reactions carried out in an N2-filled glovebox.
The catalyst's utility was further demonstrated in one-pot workflows. It was loaded onto ChemBeads (glass beads dry coated with solid catalysts or reagents by using acoustic mixing) for precise dosing in a 24-well format. After borylation, the products underwent Suzuki-Miyaura coupling, yielding biaryl products in 20 out of 24 cases. Even in the reactions where cross-coupling failed, successful borylation was observed.
The workflow was extended in a high-throughput experimentation (HTE) format, combining 12 heteroarenes with 8 aryl halides to generate 96 potential products at a 400 nmol scale. Products were observed in 81 of the 96 wells, demonstrating the system's efficiency. This approach was extended to include: C–H borylation and Ni-catalyzed alkylation, achieving 81 out of 96 successes; C–H borylation and Chan-Lam coupling, with 43 out of 96 successes; C–H borylation and bromination, yielding 17 successful cases out of 24.
[(tmphen)Ir(coe)2Cl] represents a major breakthrough in C–H functionalisation, balancing air stability, high activity, and ease of use. Its robust performance across scales and in diverse reaction conditions paves the way for broader adoption of iridium-based catalysis. Beyond borylation, the catalyst sets the stage for advancements in other catalytic transformations, offering streamlined workflows and enabling sustainable and efficient chemical synthesis.
This study underscores the transformative potential of single-component catalysts in addressing long-standing challenges, fostering innovations in both industrial processes and miniaturised experimental systems.
Photochemical Permutation of Thiazoles and Other Azoles
Thiazoles and isothiazoles are widely found in many pharmaceuticals and agrochemicals. Traditional methods for synthesising this class of heterocycles typically involve condensation reactions. In this article, the authors introduce a novel and conceptually distinct approach: the rearrangement of thiazole derivatives under mild photochemical irradiation. This methodology demonstrates a broad scope, good tolerance to various reaction conditions, and practical application in complex systems.
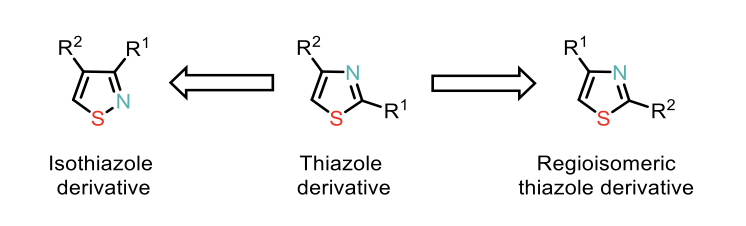
Figure 2: Structural permutation of a disubstituted thiazole derivative
The development of this methodology is based on two key strategies:
- Utilising easily accessible thiazole or isothiazole derivatives to achieve structural permutation, resulting in isomeric thiazoles (e.g., C2-substituted to C4-substituted) while preserving the heterocyclic ring.
- Completely transforming the heterocyclic core (e.g., converting thiazole to isothiazole; see Figure 2).
The underlying concept involves dearomatisation of the heterocyclic ring through photoexcitation, enabling various structural permutations. However, since simple isothiazoles and thiazoles exhibit similar absorption profiles (leading to poor selectivity), the authors employed substituted thiazoles and fine-tuned the reaction conditions to achieve selectivity.
Initial optimisation experiments involved irradiating C2-Ph-thiazole in 1,2-dichloroethane (DCE) at 310 nm at room temperature, which selectively yielded C4-Ph-thiazole. Interestingly, switching the solvent to methanol produced C3-Ph-thiazole as the major product in good yield. Further improvements in chemical yields were achieved by adding stoichiometric amounts of triethylamine.
This methodology exhibits a broad substrate scope, including di- and tri-substituted thiazoles, benzothiazoles, and other azoles such as isoxazoles (converted to oxazoles) and pyrazoles (converted to imidazoles) with good functional group tolerance (yields ranging from 22% to 89%). Its robustness was further demonstrated using complex thiazoles and azole-containing molecules, such as azoramide and VHL Ligand 1 (see Scheme 3).
The merits of this approach include direct access to a variety of isothiazole derivatives in a single transformation, avoiding de novo synthesis, and its applicability to various other azoles. Notably, the electronic effects of substituents (e.g., p-CF3 or p-OMe) did not influence permutation selectivity, as corroborated by computational studies.
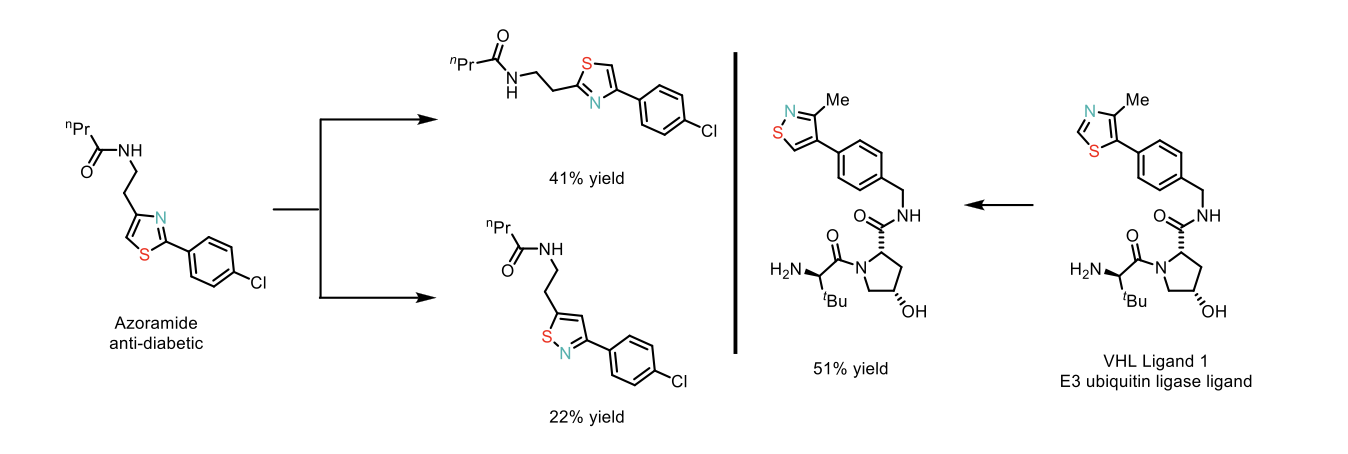
Scheme 3: Application of thiazole permutations in complex systems
This novel photochemical methodology for rearranging thiazole derivatives offers a significant breakthrough in heterocyclic chemistry. With its broad substrate scope, functional group tolerance, and ability to access diverse scaffolds under mild conditions, it provides an efficient alternative to traditional synthetic methods. Its potential applications in complex molecule synthesis makes it a valuable tool for advancing pharmaceutical and agrochemical research.
If you have an interesting synthetic or medicinal chemistry challenge you would like to discuss with Domainex’s experts, please get in touch.